Over the course of the summer, I was granted the opportunity to shadow not just one, but three distinguished laboratories at Purdue University in Indiana, led by exceptional researchers: Dr. Robert V Stahelin, a renowned expert in the field of biochemistry and the research of the Ebola Virus; Dr. Subramaniam Ramaswamy, a visionary in the realm of bio-physics; and Dr. Leopold Green, a trailblazer in the study of synthetic biology.

Over the next three weeks, I immersed myself fully in the daily activities of the PI4D Lab, which stands for Purdue Institute of Inflammation, Immunology, and Infectious Diseases. Each day was a fresh opportunity to delve into various realms of scientific exploration. The diversity of research opportunities spanned across fields such as bio-chemistry, bio-physics, synthetic biology, biological engineering, and the fascinating world of the microbiome.
I had the privilege of observing experiments involving lipid-protein interactions, which play a crucial role in understanding cellular processes and disease mechanisms, in Dr. Stahelin’s lab. Here, Virus Like Particles (VLPs) of the Ebola Virus, which are molecular assemblies that resemble viruses in structure but lack the genetic material required for replication, are used to study the seven stages of the virus replication process. These particles are engineered to mimic the outer appearance of viruses, including their shape and surface proteins, but they do not contain viral DNA or RNA. As a result, VLPs are non-infectious and pose no risk of causing disease.
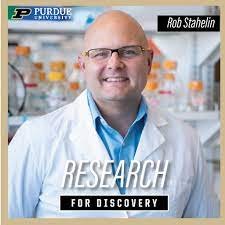
Dr. Robert V. Stahelin, a distinguished researcher in biochemistry, has made significant contributions to the study of VLPs. His work investigates the interactions between lipids and proteins within the phospholipid layer of the cell membrane and VLPs and their role in cellular processes. By understanding these interactions, researchers like Dr. Stahelin aim to gain insights into various disease mechanisms and develop potential therapeutic approaches.
VLPs have shown great promise in the field of vaccinology and drug delivery. Due to their virus-like properties, they can elicit a strong immune response without the risk of causing an actual infection. Thus, they serve as a safe and effective platform for vaccine development, offering protection against infectious diseases. Additionally, VLPs can be engineered to carry specific payloads, making them valuable tools for targeted drug delivery in treating various medical conditions. As of now, several VLP-based vaccines targeting different viruses, including Norwalk Virus, HIV, Ebola Virus, SARS-CoV-2 Virus, Respiratory Syncytial Virus (RSV), and Influenza Virus, are still under different clinical trials. In fact, a few VLP vaccines have even made it to the market, showing their commercial feasibility.
As the field of VLP research continues to evolve, Dr. Stahelin’s work and other scientists’ contributions are paving the way for innovative applications that can revolutionize medicine and disease prevention. Dr. Stahelin’s team’s dedication to uncovering the intricacies of these interactions left a profound impression on me, igniting a new fascination for the complexities of cellular biology.

On the other hand, I was mesmerized by the advanced imaging techniques and simulations employed to decipher the cell’s inner workings, giving rise to potential disease treatment and prevention breakthroughs at Dr. Subramaniam Ramaswamy’s Lab. Kanaga Vijayan, a Post Doc Student at Dr. Subramaniam Ramaswamy’s Lab, guided me around the lab, where I learnt different aspects of their research. I was introduced to the study of Macromolecular structure and function using diffraction and cryo-EM, along with the study of Enzyme mechanisms, protein-protein, and protein-ligand interactions.
X-ray diffraction is a powerful technique used to determine the three-dimensional structures of macromolecules, including proteins and nucleic acids. It involves bombarding a crystallized sample with X-rays and analyzing the resulting diffraction pattern (the pattern by which a beam of light is spread out), to reconstruct the electron density map of the molecule. This technique has been essential in explaining the structures of proteins and other biomolecules at atomic resolution, providing insights into their functions, active sites, and overall architectures.
Cryo-EM allows researchers to visualize large macromolecules and complexes, such as membrane proteins and viruses, at near-atomic resolution (imaging or analyzing structures with a level of detail that approaches the scale of individual atoms). Unlike X-ray crystallography, cryo-EM does not require crystallization, making it applicable to a wider range of molecules. In this technique, samples are rapidly frozen to preserve their native structures, and a beam of electrons is used to image the sample from different angles. Advanced image processing techniques are then used to reconstruct three-dimensional structures from the collected images.
By combining these techniques, the students and professors working in the lab gain a comprehensive understanding of macromolecular structures, their dynamic behaviors, and their roles in various biological processes. This knowledge is essential for advancing fields such as drug discovery, molecular medicine, and understanding the molecular basis of diseases.
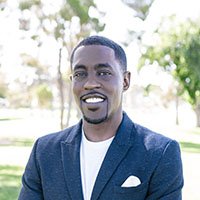
At the third lab, Dr. Leopold Green’s lab, I ventured into synthetic biology, a field brimming with endless possibilities. I learned about the Model-guided Design of a Biological Controller for Septic Wound Healing Regulation he and his student Derrik Dankwa created. They deployed an ordinary differential equation (ODE)-based model to capture the hyper and hypo-inflammatory phases of sepsis wound healing. This essentially meant that they developed a mathematical model to study how the inflammatory response evolves during the healing process of sepsis.
Sepsis is a body’s response to an infection, leading to a dysregulated immune response with hyper-inflammatory and hypo-inflammatory phases. The hyper-inflammatory phase is when the immune system becomes excessively active, and the hypo-inflammatory phase is when the immune system becomes excessively suppressed. It is crucial to balance these phases for effective wound healing and recovery.
Using the ODE-based mathematical equation to form models, researchers can simulate and analyze the temporal dynamics of the immune response during both the hyper-inflammatory and hypo-inflammatory phases of sepsis wound healing, as these equations represent the rates of change of various variables involved in the immune response, such as immune cell populations, cytokines, and other signaling molecules.
As I immersed myself in the research at Purdue University, I realized that my passion for scientific exploration had grown exponentially. I found myself wanting to pursue scientific research. Looking back on those three weeks, I am grateful for the opportunity to shadow scientists at Purdue University. The experience deepened my understanding of various scientific disciplines and fuelled my desire to pursue a career dedicated to scientific discovery. The shadowing program allowed me to witness first-hand the dedication, innovation, and teamwork that form the backbone of impactful research.
So proud of you Navya . Extensive research on this subject is an interesting read.
Keep it up . All the best !